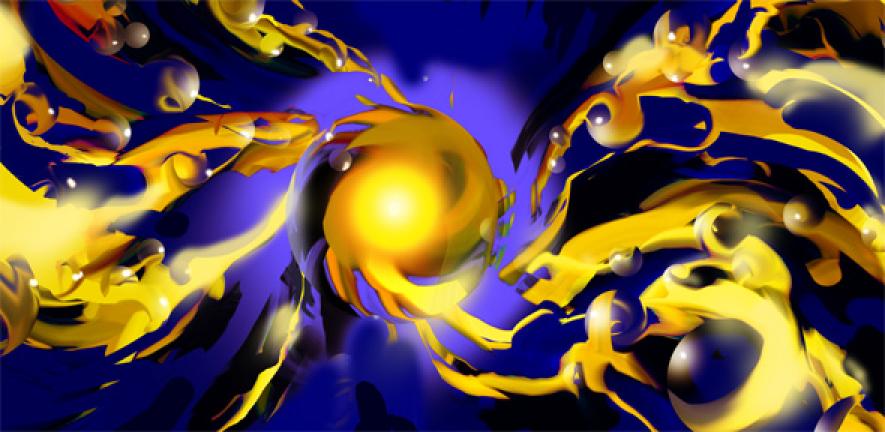
Nanotechnology is creating new opportunities for fighting disease – from delivering drugs in smart packaging to nanobots powered by the world’s tiniest engines.
Nanotechnology is creating new opportunities for fighting disease – from delivering drugs in smart packaging to nanobots powered by the world’s tiniest engines.
Designing these particles, loading them with drugs and making them clever so that they release their cargo in a controlled and precise way: it’s quite a technical challenge.
Mark Welland
Chemotherapy benefits a great many patients but the side effects can be brutal.
When a patient is injected with an anti-cancer drug, the idea is that the molecules will seek out and destroy rogue tumour cells. However, relatively large amounts need to be administered to reach the target in high enough concentrations to be effective. As a result of this high drug concentration, healthy cells may be killed as well as cancer cells, leaving many patients weak, nauseated and vulnerable to infection.
One way that researchers are attempting to improve the safety and efficacy of drugs is to use a relatively new area of research known as nanothrapeutics to target drug delivery just to the cells that need it.
Professor Sir Mark Welland is Head of the Electrical Engineering Division at Cambridge. In recent years, his research has focused on nanotherapeutics, working in collaboration with clinicians and industry to develop better, safer drugs. He and his colleagues don’t design new drugs; instead, they design and build smart packaging for existing drugs.
Nanotherapeutics come in many different configurations, but the easiest way to think about them is as small, benign particles filled with a drug. They can be injected in the same way as a normal drug, and are carried through the bloodstream to the target organ, tissue or cell. At this point, a change in the local environment, such as pH, or the use of light or ultrasound, causes the nanoparticles to release their cargo.
Nano-sized tools are increasingly being looked at for diagnosis, drug delivery and therapy. “There are a huge number of possibilities right now, and probably more to come, which is why there’s been so much interest,” says Welland. Using clever chemistry and engineering at the nanoscale, drugs can be ‘taught’ to behave like a Trojan horse, or to hold their fire until just the right moment, or to recognise the target they’re looking for.
“We always try to use techniques that can be scaled up – we avoid using expensive chemistries or expensive equipment, and we’ve been reasonably successful in that,” he adds. “By keeping costs down and using scalable techniques, we’ve got a far better chance of making a successful treatment for patients.”
In 2014, he and collaborators demonstrated that gold nanoparticles could be used to ‘smuggle’ chemotherapy drugs into cancer cells in glioblastoma multiforme, the most common and aggressive type of brain cancer in adults, which is notoriously difficult to treat. The team engineered nanostructures containing gold and cisplatin, a conventional chemotherapy drug. A coating on the particles made them attracted to tumour cells from glioblastoma patients, so that the nanostructures bound and were absorbed into the cancer cells.
Once inside, these nanostructures were exposed to radiotherapy. This caused the gold to release electrons that damaged the cancer cell’s DNA and its overall structure, enhancing the impact of the chemotherapy drug. The process was so effective that 20 days later, the cell culture showed no evidence of any revival, suggesting that the tumour cells had been destroyed.
While the technique is still several years away from use in humans, tests have begun in mice. Welland’s group is working with MedImmune, the biologics R&D arm of pharmaceutical company AstraZeneca, to study the stability of drugs and to design ways to deliver them more effectively using nanotechnology.
“One of the great advantages of working with MedImmune is they understand precisely what the requirements are for a drug to be approved. We would shut down lines of research where we thought it was never going to get to the point of approval by the regulators,” says Welland. “It’s important to be pragmatic about it so that only the approaches with the best chance of working in patients are taken forward.”
The researchers are also targeting diseases like tuberculosis (TB). With funding from the Rosetrees Trust, Welland and postdoctoral researcher Dr Íris da luz Batalha are working with Professor Andres Floto in the Department of Medicine to improve the efficacy of TB drugs.
Their solution has been to design and develop nontoxic, biodegradable polymers that can be ‘fused’ with TB drug molecules. As polymer molecules have a long, chain-like shape, drugs can be attached along the length of the polymer backbone, meaning that very large amounts of the drug can be loaded onto each polymer molecule. The polymers are stable in the bloodstream and release the drugs they carry when they reach the target cell. Inside the cell, the pH drops, which causes the polymer to release the drug.
In fact, the polymers worked so well for TB drugs that another of Welland’s postdoctoral researchers, Dr Myriam Ouberaï, has formed a start-up company, Spirea, which is raising funding to develop the polymers for use with oncology drugs. Ouberaï is hoping to establish a collaboration with a pharma company in the next two years.
“Designing these particles, loading them with drugs and making them clever so that they release their cargo in a controlled and precise way: it’s quite a technical challenge,” adds Welland. “The main reason I’m interested in the challenge is I want to see something working in the clinic – I want to see something working in patients.”
Could nanotechnology move beyond therapeutics to a time when nanomachines keep us healthy by patrolling, monitoring and repairing the body?
Nanomachines have long been a dream of scientists and public alike. But working out how to make them move has meant they’ve remained in the realm of science fiction.
But last year, Professor Jeremy Baumberg and colleagues in Cambridge and the University of Bath developed the world’s tiniest engine – just a few billionths of a metre in size. It’s biocompatible, cost-effective to manufacture, fast to respond and energy efficient.
The forces exerted by these ‘ANTs’ (for ‘actuating nano-transducers’) are nearly a hundred times larger than those for any known device, motor or muscle. To make them, tiny charged particles of gold, bound together with a temperature-responsive polymer gel, are heated with a laser. As the polymer coatings expel water from the gel and collapse, a large amount of elastic energy is stored in a fraction of a second. On cooling, the particles spring apart and release energy.
The researchers hope to use this ability of ANTs to produce very large forces relative to their weight to develop three-dimensional machines that swim, have pumps that take on fluid to sense the environment and are small enough to move around our bloodstream.
Working with Cambridge Enterprise, the University’s commercialisation arm, the team in Cambridge's Nanophotonics Centre hopes to commercialise the technology for microfluidics bio-applications. The work is funded by the Engineering and Physical Sciences Research Council and the European Research Council.
“There’s a revolution happening in personalised healthcare, and for that we need sensors not just on the outside but on the inside,” explains Baumberg, who leads an interdisciplinary Strategic Research Network and Doctoral Training Centre focused on nanoscience and nanotechnology.
“Nanoscience is driving this. We are now building technology that allows us to even imagine these futures.”
Read more about research on future therapeutics in Research Horizons magazine.
The text in this work is licensed under a Creative Commons Attribution 4.0 International License. For image use please see separate credits above.