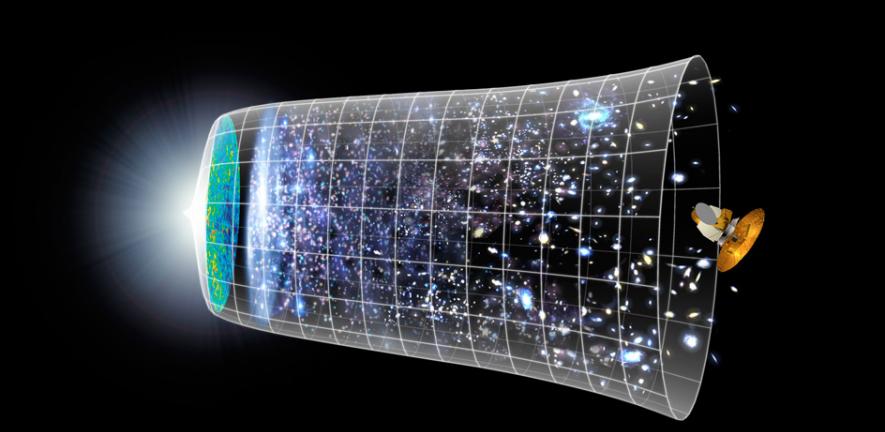
New research is bringing astronomers closer to unlocking the ‘dark ages’ of the cosmos.
New research is bringing astronomers closer to unlocking the ‘dark ages’ of the cosmos.
One of the most exciting opportunities offered by these observations is the possibility to go even further back in time than the epoch of reionisation, to a few minutes after the Big Bang.
Professor Max Pettini
Astronomers can now look back in time over almost all of the 13.7-billion-year history of the universe, from the present epoch to when the universe was still in its infancy, thanks to ever-more powerful telescopes that detect light reaching us from billions of light-years away. But one observational frontier still remains – a period lasting perhaps less than half a billion years after the Big Bang that astronomers refer to as the ‘dark ages.’
One of the many questions about this time is how our universe evolved from one consisting only of the two simplest elements, hydrogen and helium, into one that boasts the wonderful mix of elements that make up our familiar world, such as carbon, oxygen and iron.
A new understanding of this process has been made possible by the work of Professor Max Pettini and colleagues at Cambridge’s Institute of Astronomy (IoA). To do this, they have turned to the most luminous objects in the universe, quasars, using the light they emit as a tool to pick out the characteristics of what lies between us and the quasar – rather like a torch being shone through the universe.
Written in the stars
What makes the dark ages of the universe opaque to astronomical telescopes are the clouds of neutral hydrogen and helium gas that were formed when temperatures had cooled enough for protons and electrons created at the Big Bang to recombine. The epoch ended when dense areas of gas collapsed in on themselves to form the first stars – massive, intensely hot, cosmic ‘ovens’ of nuclear fusion. Astronomers believe that the ultraviolet light these primitive stars emitted caused the gas in their surroundings to ionise, with the result that the universe became increasingly transparent to optical and infrared telescopes. This was the `epoch of reionisation’, which eventually put an end to the cosmic dark ages.
Too unstable to last longer than a few million years, and dying in spectacular supernova explosions, the early stars were to leave a legacy – they began the chemical evolution of the cosmos. They seeded further generations of stars (including some that we still see today) with the chemical elements synthesised in their hot interiors from the primordial hydrogen and helium.
‘Computer simulations have painted a broad-brush picture of what we understand occurred during the transition from the dark ages, prior to reionisation, to today’s rich tapestry of galaxies and intergalactic matter. But only experimental observations with telescopes can fill the many gaps still present in our knowledge of this cosmic evolution,’ explains Pettini. ‘The difficulty is how to observe what is now unobservable – the first stars no longer exist and the earliest observable galaxies are several generations younger.’
The solution has been to look indirectly at the remnants left by the earliest stars using a technique known as Quasar Absorption Line Spectroscopy. The technique was pioneered in the mid-1970s by, among others, Professor Alec Boksenberg (Pettini’s PhD supervisor), formerly at University College London and now at the IoA, and Professor Wallace Sargent at the California Institute of Technology in Pasadena. The latest research from the Pettini group represents the first time the technique has been used to identify a chemical imprint of the earliest stars to be born after the Big Bang.
Missing link
Quasars are black holes with the mass of a billion suns. Although the black hole itself emits no light, the force of its gravity is so huge that any matter around it falls into the quasar, and in so doing emits radiation intense enough that astronomers can detect it on Earth tens of billions of years after it was emitted.
‘The light that quasars emit provides a backdrop against which any gas cloud in the path of their light – whether in galaxies or in between galaxies – can be measured,’ says Pettini. What interests Pettini and his collaborators is the gas that is closest to the primordial composition. Such gas may still bear traces of the first chemical elements manufactured by the first stars and released into their surroundings when they exploded, in effect providing a fossil record of the earliest events in the chemical evolution of the universe.
Taking precision measurements with sufficient spectral resolution of the properties of this gas has required use of the world’s largest telescopes in Hawaii and Chile. Such instruments provide the means to measure accurately the relative proportions of hydrogen (created in the Big Bang) and elements assembled subsequently in the interiors of stars.
Among the different types of gas clouds that can produce absorption lines in quasar spectra, the team have learnt to recognise clouds called ‘damped Lyman alpha systems’ (DLAs) that are most likely to be associated with galaxies that are still at an early stage of evolution. And they can now identify, among the thousands of DLAs known, the rare few that have undergone a very small amount of star formation.
Cosmic clouds
The latest results of this five-year programme have just been published in the journal Monthly Notices of the Royal Astronomical Society by Ryan Cooke, Max Pettini and Regina Jorgenson working at the IoA, together with Charles Steidel and Gwen Rudie at the California Institute of Technology in Pasadena.
The authors report the first analysis of the composition of a near-primordial gas cloud containing the elements made by a star that exploded perhaps as many as 13 billion years ago.
Specifically, the tiny amounts of elements present in the cloud are in very unusual proportions, very different from their relative proportions in normal stars today, like our Sun. Most significantly, the ratio of carbon to iron is 35 times greater than measured in the Sun. Such an overabundance of carbon relative to iron – as well as the relative proportions of other elements including nitrogen, oxygen, aluminium, silicon and sulphur – match closely computer calculations of the elements synthesised and released by a star 25 times more massive than the Sun and originally consisting of only hydrogen and helium.
Weighing the universe
Moving towards a new understanding of the earliest stars isn’t the only outcome of the research. ‘In my view, one of the most exciting opportunities offered by these observations is the possibility to go even further back in time than the epoch of reionisation, to a few minutes after the Big Bang, when the whole universe resembled a star and was hot enough to create elements such as deuterium (a heavier form of hydrogen) and helium out of pure hydrogen,’ says Pettini.
It turns out that the amount of deuterium manufactured in the Big Bang depends sensitively on the total amount of ordinary matter present in the Universe. As a result, Pettini has been able to ‘weigh’ the mass of the entire Universe by measuring the ratio of deuterium to hydrogen in the most pristine DLAs. The answer, which agrees beautifully with other, independent, measures, is that the universe is 4% ordinary matter, 22% dark matter and 74% dark energy.
‘For the first time in the history of mankind, scientists are able to determine fundamental cosmological numbers that describe our universe with precision,’ comments Pettini. ‘But we have yet to understand why these proportions are as they are and, most fundamentally, the underlying physics that determines these fractions.’
Nevertheless, the discovery of what Pettini and colleagues consider the missing link between the oldest stars still in existence in the Milky Way and the first generation of stars after the Big Bang brings us closer to unlocking the dark ages of the cosmos. One day, Pettini hopes that a DLA may even be discovered that has no metals – in other words, a cosmic cloud left over from a time even before the first stars.
For more information, please contact Professor Max Pettini (pettini@ast.cam.ac.uk) at the Institute of Astronomy. Professor Pettini has recently been elected a fellow of the Royal Society and awarded the Royal Astronomical Society Herschel Medal for his seminal contributions to extragalactic astronomy.
This work is licensed under a Creative Commons Licence. If you use this content on your site please link back to this page.